舞茸蛋白多糖和维生素C联合使用诱导肾细胞癌的
日期:2019-07-31 / 人气:
声明:本文摘自Intergrative Cancer Therapies 12 (5) 442 –448(2013年), 即《癌症综合治疗》第12卷第5期,是由美国SAGE出版公司出版发行的一本国际性癌症治疗杂志。该杂志为季刊,每年3、6、9、12月各出版一期。Intergrative Cancer Therapies 关注癌症治疗领域的新动向,特别是替代医学和传统医学与常规治疗方法的有效结合,癌症的综合疗法包括饮食、生活方式、运动、减压及营养补充等方面的治疗性干预,以及实验疫苗、慢性化疗等其他新疗法,杂志为读者提供严谨的临床研究,病例报道,文献综述等文章。为方便广大读者阅读,我们对论文摘要进行了中文翻译,译文仅供参考,如有不足之处,敬请指正。
【参考译文】
Induction of Cell Death in Renal Cell Carcinoma With Combination of D-Fraction and Vitamin C
舞茸蛋白多糖和维生素C联合使用诱导肾细胞癌的细胞死亡
摘 要
假设:尽管晚期肾细胞癌(RCC)有多种传统的治疗方法可供选择,但是效果都差强人意,这就需要找到更有效的方法。一种具有生物活性的舞茸蛋白多糖(PDF)显示出抗癌与调节免疫的活性,且维生素C能增强其效果。我们假设PDF和VC联合使用能作为替代疗法更有效地抑制RCC的生长。
试验设计:我们根据剂量大小测试了PDF+VC对RCC细胞生存率的影响,同时进行生物化学试验来探索其生长调节机制。
方法:用不同浓度的PDF和VC及两者的混合物处理人类肾细胞癌(RCC)ACHN细胞系。在指定的时间用MTT法测定细胞生存率,同时进行脂质过氧化反应试验、细胞周期分析以及蛋白免疫印迹分析等试验。
结果:单独使用时,当PDF浓度>500μg/ml,VC浓度≥300μM时,试验进行72小时后两者均能显著降低细胞生存率。对PDF和VC的不同浓度组合进行测试,单独时的无效浓度PDF300μg/ml与VC200μM联合使用时在试验进行24小时后能达到90%的细胞死亡率。脂质过氧化反应试验分析结果显示这个组合能显著提升氧化应激反应(约2.5倍)。细胞周期分析也显示在试验6小时后出现了G1细胞周期阻滞。蛋白免疫印迹法进一步表明Bcl2蛋白的减少,这提示细胞凋亡。
结论:此项试验显示PDF和VC联合使用后有较高的细胞毒性,能显著诱导ACHN细胞死亡。这种细胞毒性机制主要是由氧化应激导致,并伴随有G1细胞周期停滞。细胞凋亡调节蛋白(Bcl2,Bax,PARP)的变化提示这种由PDF和VC诱导的细胞死亡很可能与细胞凋亡有关。因此PDF和VC协同作用诱导细胞死亡,可能作为一种替代疗法具有临床意义,能改善晚期RCC的治疗方案。
【论文原文】
Integrative Cancer Therapies
12(5) 442 –448
© The Author(s) 2013
Reprints and permissions:
sagepub.com/journalsPermissions.nav
DOI: 10.1177/1534735412473643
ict.sagepub.com
Induction of Cell Death in Renal Cell Carcinoma With
Combination of D-Fraction and Vitamin C
Bobby Alexander, MD1, Andrew I. Fishman, MD1, Majid Eshghi, MD1,
Muhammad Choudhury, MD1, and Sensuke Konno, PhD1
Abstract
Hypothesis. Although several conventional therapeutic options for advanced renal cell carcinoma (RCC) are currently available, the unsatisfactory outcomes demand establishing more effective interventions. D-fraction (PDF), a bioactive proteoglucan of Maitake mushroom, demonstrates anticancer and immunomodulatory activities, which are also shown to be potentiated by vitamin C (VC). We thus hypothesized that a combination of PDF and VC (PDF + VC) could be an alternative approach to more effectively inhibit the growth of RCC. Study design. We examined the dose-dependent effects of PDF + VC on RCC cell viability and also performed biochemical assays to explore the growth regulatory mechanism. Methods. Human RCC, ACHN cell line, was employed and exposed to varying concentrations of PDF or VC and their combinations. Cell viability at specified times was determined by MTT assay. Lipid peroxidation assay, cell cycle analysis, and Western blot analysis were also performed. Results. PDF or VC alone led to the significant reduction in cell viability at 72 hours with PDF >500 µg/mL and VC ≥300 µM. When various combinations of PDF and VC were tested, the combination of the ineffective concentrations of PDF (300 µg/mL) and VC (200 µM) resulted in ~90% cell death in 24 hours. Lipid peroxidation assay then indicated significantly (~2.5 fold) elevated oxidative stress with this PDF + VC. Cell cycle analysis also indicated a G1 cell cycle arrest following a 6-hour PDF + VC treatment. Western blots further revealed a downregulation of Bcl2, an upregulation of Bax, and proteolytic activation of PARP (poly[ADP-ribose] polymerase) in PDF + VC-treated cells, indicating induction of apoptosis. Conclusion. The present study demonstrates that the combination of PDF and VC can become highly cytotoxic, inducing severe cell death in ACHN cells. This cytotoxic mechanism appears to be primarily attributed to oxidative stress, accompanied by a G1 cell cycle arrest. Such cell death induced by PDF + VC could be more likely linked to apoptosis, as indicated by the modulation of apoptosis regulators (Bcl2, Bax, and PARP). Therefore, as PDF and VC may work synergistically to induce apoptotic cell death, they may have clinical implications in an alternative, improved therapeutic modality for advanced RCC.
Keywords
D-fraction, vitamin C, oxidative stress, cell death, apoptosis regulators, renal cell carcinoma
Introduction
As the incidence of renal cell carcinoma (RCC) has risen steadily for the past 3 decades in the United States,1 radio-graphic images became widely available and revolutionized the diagnosis of RCC. Still, nearly 30% of these patients would present with metastatic disease at the time of diagnosis.2 Although the primary treatment for localized RCC is surgical resection (nephrectomy), 30% to 40% of patients would have a recurrence leading subsequently to metastatic disease.3,4 The prognosis of those with metastatic RCC is poor, and its management/treatment is limited, resulting in a median survival of 6 to 12 months.5,6 Such a high rate of metastatic potential of RCC is a major concern, and establishing more effective surgical and medical treatments is urgently demanded.7
Few reliable, effective treatments for metastatic RCC are currently available, because several strategies attempted in the past were rather ineffective and unsuccessful in the adjuvant setting, including surgical removal of distant metastasis, radiation therapy, chemotherapy, and immunotherapy.2,3 Only immunotherapy with interferon-α and/or interleukin-2 was somewhat effective thus far, having response rates of 15% to 25% in trials.8,9 Yet, this is far from the satisfactory results we are aiming for.
Recent advances in understanding the genetics, biochemistry, and molecular biology of RCC have led to various novel targeted treatments to attain higher response rates.7,10 Those include allogeneic stem cell transplantation,11 tumor vaccines,12 or targeting tumor antigens13,14 and specific biochemical pathways.15 For instance, nonmyeloablative stem cell transplantation involves an infusion of a peripheral blood stem cell allograft from an HLA (human leukocyte antigen)-identical sibling.11 Tumor vaccines may help enhance host immunity using therapies such as autologous or donor dendritic cells.12 Targeting antigens involve chimeric monoclonal antibody, G250, recognizing CA-IX antigen abundantly expressed in the clear cell type of RCCs13 and another antibody, bevacizumab, targeting VEGF to inhibit tumor angiogenesis.14 Moreover, certain
agents and antibodies could be used to target specific signal transduction pathways (PI3K/AKT, Ras/Raf/MEK, etc).15 However, all these innovative approaches are yet investigational or experimental and their actual efficacy needs to be properly assessed through further studies and trials.
Meanwhile, we were interested in exploring an unconventional but more effective therapeutic modality for RCC using natural agents/substances, although sufficient scientific studies have not been performed on most of them. Yet several studies scientifically demonstrated biological significance of some natural agents. Particularly, one of the medicinal mushrooms, Maitake (Grifola frondosa), has been extensively studied.16 Its antitumor activity was demonstrated in tumor-bearing mice,17 through activation of various immune effectors including macrophages, cytotoxic T-lymphocytes, natural killer cells, and so on.18 Recently, induction of apoptosis by this Maitake extract (D-fraction) was also reported in breast cancer cells.19 Such a bioactive component of Maitake extract has been identified as β-glucan (proteoglucan), a protein-bound polysaccharide, with a molecular weight of ~1 × 106 Da.16 Accordingly, it was of our interest to investigate whether this mushroom β-glucan might have a growth inhibitory effect on an RCC model in vitro, as a possible complementary and alternative approach.
Materials and Methods
Cell Culture
The human renal carcinoma ACHN cells were maintained in RPMI-1640 medium containing 10% fetal bovine serum, penicillin (100 units/mL), and streptomycin (100 µg/mL). Standardized D-fraction (PDF) was a gift from the manufacturer (Mushroom Wisdom, Inc). For experiments, ACHN cells were seeded at the initial cell density of 2 × 105 cells/mL in 6-well plates or T-75 flasks and cultured with varying concentrations of PDF or in combination with vitamin C (VC) for specified times.
Cell Viability Assay
Cell viability was determined by MTT (3-[4,5-dimethylthiazol-2-yl]-2,5-diphenyl-tetrazolium bromide) assay following the vendor’s protocol (Sigma-Aldrich, St Louis, MO) with minor modifications. Briefly, at the harvest time, MTT reagent (0.5 mg/mL) was added to all wells in the
6-well plate, which was then incubated for 3 hours. After the MTT reagent was removed from all wells, DMSO (dimethyl sulfoxide) was added to each well to dissolve the formazan crystals. Absorbance of fomazan solution was read in a microplate reader, and cell viability was expressed
as percentage of viable cell number relative to the control reading (100%).
Lipid Peroxidation (LPO) Assay
Possible damage in the plasma membrane under oxidative stress was assessed by LPO assay measuring the formation of malondialdehyde (MDA), an end product from peroxidation of polyunsaturated fatty acids in the plasma membrane.20 The detailed procedures were described in the vendor’s protocol (Calbiochem, Albuquerque, NM), and the amount of MDA formed was expressed in micromolar concentrations determined from the MDA standards.
Cell Cycle Analysis
A BD FACScan flow cytometer (Franklin Lakes, NJ), equipped with a double discrimination module, was employed for cell cycle analysis. Approximately 1 × 106 cells were resuspended in 500 µL of propidium iodide solution (containing 20 µg/mL propidium iodide, 0.2 mg/mL RNase, 0.2 mg/mL EDTA, 0.5% NP-40) and incubated at room temperature for 1 hour. Ten thousand nuclei were analyzed for each sample, and CellFit software (BD) was used to quantify cell cycle compartments and estimate cell cycle phase fractions.
Western Blot Analysis
The procedures essentially followed the protocol described previously.21 Briefly, cell lysates were obtained from control and agent-treated cells by “freeze-thaw” in liquid nitrogen. An equal amount of cell lysates (7 µg) was first subjected to 10% SDS-polyacrylamide gel electrophoresis and then transferred to a nitrocellulose membrane. The blot (membrane) was incubated for 90 minutes with the primary antibodies against Bcl2, Bax, or poly(ADP-ribose) polymerase (PARP;
Santa Cruz Biotechnology, Santa Cruz, CA), followed by a 30-minute incubation with the secondary antibody conjugates. The specific immunoreactive protein bands were then detected by chemiluminescence following the manufacturer’s protocol (KPL, Gaithersburg, MD).
Statistical Analysis
All data were presented as mean ± SD (standard deviation), and statistical differences between groups were assessed with either 1-way analysis of variance (ANOVA) or the unpaired Student’s t test. Values of P < .05 were considered to indicate statistical significance.
Results
Effects of PDF or VC on ACHN Cell Viability
ACHN cells were cultured with varying concentrations of PDF (0-1000 µg/mL) or VC (0-700 µM), and cell viability was determined at 72 hours. PDF was capable of inducing ~40% and ~65% reduction in cell viability at 700 and 1000 µg/mL, respectively (Figure 1A). VC also showed significant viability reduction as its concentrations increased, resulting in ~16%, ~52%, and >90% viability reduction at 300, 500 and 700 µM, respectively (Figure 1B). These results suggest that both PDF and VC could exhibit the cytotoxic effects on ACHN cells at their relatively high concentrations.
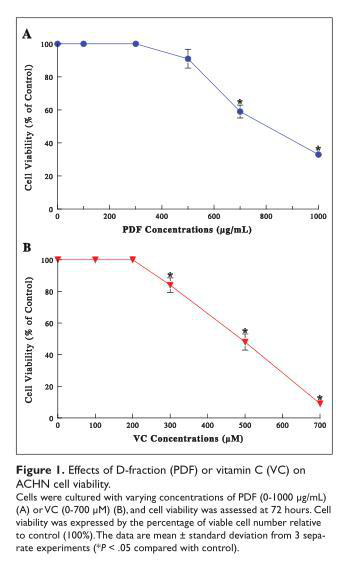
Synergistic Cytotoxic Effect of Combination of PDF and VC
As it has been postulated that VC could modulate the bioactivity of β-glucan including PDF,22 this possibility was tested next. We examined if the cytotoxic effect (on ACHN cells) could be potentiated with various combinations of PDF and VC. Interestingly, such studies revealed that severe (~90%) cell death was attained with the specific combination of 300 µg/mL PDF and 200 µM VC in 24 hours (Figure 2). Since neither 300 µg/mL PDF nor 200 µM VC alone had any effects (Figure 1), both agents must have worked synergistically to become highly cytotoxic, inducing such profound cell death.
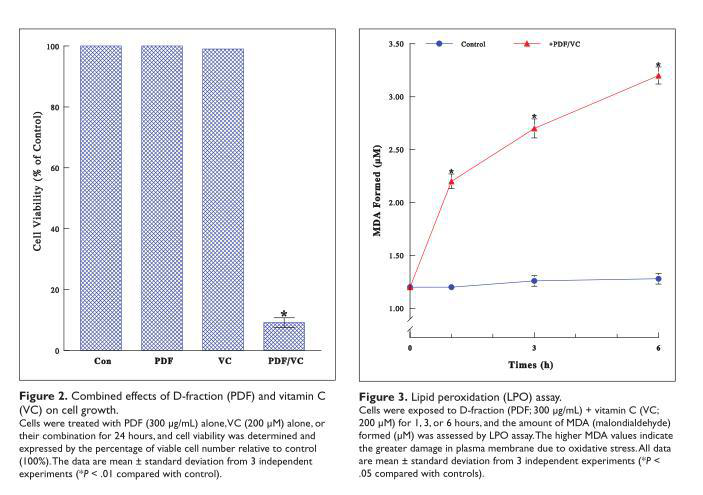
Oxidative Stress Exerted by PDF and VC Combination (PDF + VC)
To understand how a specific combination of PDF (300 µg/mL) and VC (200 µM) would become highly cytotoxic, we examined the possible involvement of oxidative stress. As we observed cell blebbing (vesicle formation) preceding cell death induced by this PDF + VC combination, it was indicative of the cells under oxidative stress. LPO assay was then performed to test this possibility, by measuring the amount of malondialdehyde (MDA) formed in the plasma membrane due to oxidative stress.20 The MDA levels following PDF + VC treatment rose significantly with time; for instance, the MDA level after 6-hour PDF+VC exposure was ~2.5-fold greater than that in controls, indicating extensive plasma membrane damage through oxidative stress (Figure 3). Thus, these results illustrate that PDF + VC can exert oxidative stress on ACHN cells, ultimately leading to cell death.
To confirm such PDF + VC-mediated oxidative stress, some common free radical scavengers, including reduced glutathione (GSH; 500 µM), catalase (CTL; 1 unit/mL), superoxide dismutase (SOD; 100 units/mL), or mannitol (Mann; 20 mM), were tested to determine if they might be able to prevent cell death by abolishing oxidative assault. Cells were cultured with PDF (300 µg/mL) + VC (200 µM) in the presence of these scavengers for 24 hours and cell viability was assessed. Both GSH and CTL were capable of effectively (>90%) preventing cell death, whereas SOD or Mann were ineffective (Table 1). Since GSH and CTL are known hydrogen peroxide (H2O2) scavengers, this finding confirms that PDF + VC cytotoxicity is primarily attributed to oxidative stress (via H2O2).

Effects of PDF + VC on Cell Cycle
To explore how oxidative stress exerted by PDF + VC would ultimately lead to cell death, cell cycle analysis was performed to unveil the early cellular event that had been modulated by PDF + VC. After cells were cultured with or without combination of PDF (300 µg/mL) and VC (200 µM)
for 6 hours, cell cycle analysis was performed. Compared with control cells, those exposed to PDF + VC displayed a 60% decrease in the S-phase cell number with a concomitant 42% increase in the G1-phase cells (Figure 4). This accumulation of cells in the G1 phase, due to a blockade of the cells entering from the G1 to the S phase, is known as a G1 cell cycle arrest that subsequently results in a growth cessation. Thus, it is plausible that such a disruption of cell cycle with PDF + VC would cause growth inhibition that leads to ultimate cell death.
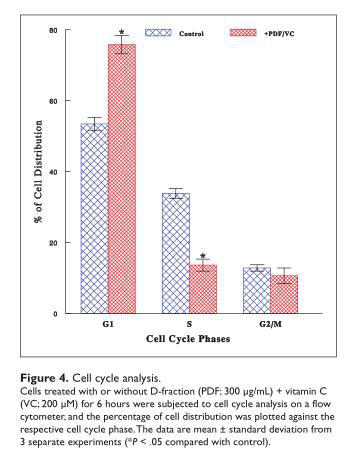
Effects of PDF + VC on Apoptosis Regulators
We further explored if PDF + VC-induced cell death would be linked to apoptosis (programmed cell death). Cells were treated with or without PDF + VC for 12 hours and analyzed for expressions of 3 key apoptosis regulators on Western blots. Such study revealed that PDF + VC treatment led to a downregulation (reduced expression) of antiapoptotic Bcl2, an upregulation (elevated expression) of pro-apoptotic Bax, and proteolytic activation (through degradation) of (Figure 5). Thus, the modulation of these specific regulators with PDF + VC suggests that cell death induced by this combination may follow apoptosis.
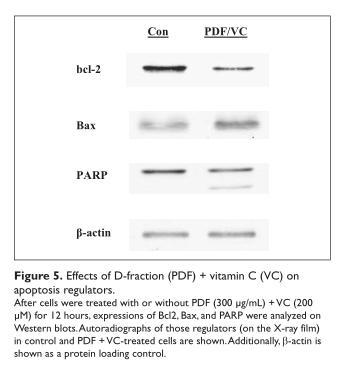
Discussion
In search for a safer, more effective treatment for RCC, with its high recurrence rate, we investigated the potential effects of a combination of mushroom proteoglucan (PDF) and vitamin C (VC) on the growth of human renal carcinoma ACHN cells. PDF and VC, each when applied by
itself, led to the significant reduction in cell viability at relatively high concentrations (Figure 1). However, when the various concentrations of PDF and VC were combined, the specific combination of ineffective PDF (300 µg/mL) and VC (200 µM) was capable of inducing severe (~90%) cell death (Figure 2). This was thus indicative of a synergistic potentiation of PDF + VC becoming highly cytotoxic to ACHN cells.
To gain an insight into PDF + VC cytotoxicity, the possible role/involvement of oxidative stress was examined by performing LPO assay and employing certain antioxidants. LPO assay clearly indicated that PDF + VC could extensively exert oxidative stress on ACHN cells (Figure 3). In addition, among several antioxidants tested, both GSH and CTL (known as H2O2 scavengers) were capable of almost completely preventing cell death induced by such oxidative stress (Table 1), implying that H2O2 could be specifically responsible for ACHN cell death. Thus, oxidative stress appears to account for the primary cytotoxic mechanism of PDF + VC.
However, oxidative stress (via H2O2) exerted by PDF + VC in this study may raise some concern for their potential clinical or therapeutic utility, because H2O2 or other free radicals could cause nonspecific, random damage to normal as well as malignant cells. Yet, it has been shown that malignant cells were more vulnerable to H2O2 (or other free radicals) than normal cells.23 The reasons for such a selective or differential effect of H2O2 remain unclear, but several evidences suggest that it may result from the inherent difference in tissue-specific antioxidant enzymes. For example, CTL and glutathione peroxidase (detoxifying H2O2 to H2O) have been shown to often be deficient or to have significantly lower activities in malignant tissues than in normal tissues.24 Moreover, reduced expressions of CTL and SOD have been also found in prostate cancer specimens, compared with those in normal or benign prostatic hyperplasia specimens.25 It thus is plausible that a difference in antioxidant enzyme activity between normal (nonmalignant) and malignant cells may account for their different susceptibility to H2O2. In the present study, it is thus possible that the amount of H2O2 generated by PDF + VC could be sufficiently cytotoxic to kill cancer (ACHN) cells (due to a low antioxidant enzyme activity) but would not be cytotoxic to
or even harm normal cells (due to a high enzyme activity). More studies are required for the role of antioxidant enzymes in cytotoxic action of PDF + VC.
Prior to cytotoxic cell death induced by PDF + VC, a G1 cell cycle arrest could be a prerequisite for a growth cessation, ultimately leading to cell death. In fact, such cell death appears to follow apoptosis, indicated by uniquely modulated expressions of apoptosis regulators (Bcl2, Bax, and PARP; Figure 5). It is then possible that oxidative stress exerted by PDF + VC could primarily interfere with the cell cycle and induce apoptosis. Nevertheless, as the efficacy of PDF + VC must still be assessed on actual RCC cases, our next study will focus on the PDF + VC effects on animals bearing RCC (in vivo).
It is also worthwhile mentioning the clinical relevance of PDF and VC. The US Food and Drug Administration has exempted PDF from a phase I study of toxicology and also approved it for the Investigational New Drug application for a phase II pilot study on advanced cancer patients.26 Although the physiologically achievable concentration of PDF has not been established at present, a daily oral intake of 60 mg PDF is currently recommended/used in the volunteer-based clinical trials. It is certainly interesting to perform an animal study to assess how much this 60 mg PDF intake would be close to “300 µg/mL” used in this study. On the other hand, a recent phase I clinical study showed that the intravenous infusion of mega-dose VC in cancer patients reached plasma concentrations greater than 10 mM (without any adverse effects).27 This indicates that “200 µM VC”(in vitro) can be indeed physiologically achievable. Thus, both PDF and VC appear to be practical and promising agents that show potential in treatment of RCC.
Conclusion
The present study demonstrates that combination of PDF and VC can be synergistically potentiated to become highly cytotoxic to ACHN cells, resulting in severe cell death. Such cytotoxicity is primarily attributed to oxidative stress accompanied by a G1 cell cycle arrest that could ultimately lead to apoptosis. Therefore, PDF and VC may have clinical implications in an alternative, improved, and safer therapeutic modality for advanced RCC. Further studies are warranted.
Acknowledgment
We thank Mr Mike Shirota (Mushroom Wisdom, Inc) for generously providing us the D-fraction used in this study.
Declaration of Conflicting Interests
The authors declared no potential conflicts of interest with respect to the research, authorship, and/or publication of this article.
Funding
The authors received no financial support for the research, author-
ship, and/or publication of this article.
References
1. Jemal A, Murray T, Ward E, et al. Cancer statistics 2005. CA Cancer J Clin. 2005;55:10-30.
2. Jacobsohn KM, Wood CG. Adjuvant therapy for renal cell carcinoma. Semin Oncol. 2006;33:576-582.
3. Glaspy JA. Therapeutic options in the management of renal cell carcinoma. Semin Oncol. 2002;29:41-46.
4. Strohmaier WL. New treatment modalities: the urologist’s view. Anticancer Res. 1999;19:1605-1609.
5. Campbell SC, Flanigan RC, Clark JI. Nephrectomy in metastatic renal cell carcinoma. Curr Treat Options Oncol. 2003;4:363-372.
6. Flanigan RC, Campbell SC, Clark JI, Picken NM. Metastatic renal cell carcinoma. Curr Treat Options Oncol. 2003;4:385-390.
7. Cohen HT, McGovern FJ. Renal cell carcinoma. N Engl J Med. 2005;353:2477-2490.
8. Motzer RJ, Murphy BA, Bacik J, et al. Phase III trial of interferon alfa-2 with or without 13-cis-retinoic acid for patients with advanced renal cell carcinoma. J Clin Oncol. 2000;18:2972-2980.
9. Yang JC, Sherry RM, Steinberg SM, et al. Randomized study of high-dose and low-dose interleukin-2 in patients with metastatic renal cell cancer. J Clin Oncol. 2003;21:3127-3132.
10. Drucker BJ. Renal cell carcinoma: current status and future prospects. Cancer Treat Rev. 2005;31:536-545.
11. Childs R, Drachenberg D. Allogeneic stem cell transplantation for renal cell carcinoma. Curr Opin Urol. 2001;11:495-502.
12. Su Z, Dannull J, Heiser A, et al. Immunological and clinical responses in metastatic renal cancer patients vaccinated with tumor RNA-transfected dendritic cells. Cancer Res. 2003;63:2127-2133.
13. Bleumer I, Oosterwijk E, Oosterwijk-Wakka JC, et al. A clinical trial with chimeric monoclonal antibody WX-G250 and low dose interleukin-2 pulsing scheme for advanced renal cell carcinoma. J Urol. 2006;175:57-62.
14. Yang JC, Haworth L, Sherry RM, et al. A randomized trial of bevacizumab, an anti-vascular endothelial growth factor antibody, for metastatic renal cancer. N Engl J Med. 2003;349:427-434.
15. Patel PH, Chaganti RSK, Motzer RJ. Targeted therapy for metastatic renal cell carcinoma. Br J Cancer. 2006;94:614-619.
16. Mizuno T, Zhuang C. Maitake, Grifola frondosa: pharmacological effects. Food Rev Int. 1995;11:135-149.
17. Hishida I, Nanba H, Kuroda H. Antitumor activity exhibited by orally administered extract from fruit body of Grifola frondosa (Maitake). Chem Pharm Bull (Tokyo). 1988;36:1819-1827.
18. Adachi K, Nanba H, Kuroda H. Potentiation of host-mediated antitumor activity in mice by β-glucan obtained from Grifola frondosa (maitake). Chem Pharm Bull (Tokyo). 1987;35:262-270.
19. Soares R, Meireles M, Rocha A, et al. Maitake (D-fraction) mushroom extract induces apoptosis in breast cancer cells by BAK-1 gene activation. J Med Food. 2011;14:563-572.
20. Dargel R. Lipid peroxidation: A common pathogenetic mechanism? Exp Toxic Pathol. 1992;44:169-181.
21. Mordente JA, Konno S, Chen Y, Wu JM, Tazaki H, Mallouh C. The effects of brefeldin A (BFA) on cell cycle progression involving the modulation of the retinoblastoma protein (pRB) in PC-3 prostate cancer cells. J Urol. 1998;159: 275-279.
22. Morishige F. The role of vitamin C in tumor therapy (human). In: Meyskens FI Jr, Parasad KN, eds Vitamins and Cancer: Human Cancer Prevention by Vitamins and Micronutrients.
Clifton, NJ: Humana Press; 1986:399-427.
23. Leung PY, Miyashita K, Young M, Tsao CS. Cytotoxic effect of ascorbate and its derivatives on cultured malignant and nonmalignant cell lines. Anticancer Res. 1993;13:475-480.
24. Sinha BK, Mimnaugh EG. Free radicals and anticancer drug resistance: Oxygen free radicals in the mechanisms of drug cytotoxicity and resistance by certain tumors. Free Radic Biol Med. 1990;8:567-581.
25. Baker AM, Oberley LW, Cohen MB. Expression of antioxidant enzymes in human prostatic adenocarcinoma. Prostate.1997;32:229-233.
26. Maitake Products Inc. Maitake D-Fraction Obtained IND for Clinical Study. Ridgefield Park, NJ: Maitake Products Inc; 1998.
27. Hoffer L, Levine M, Assouline S, et al. Phase I clinical trial of i.v. ascorbic acid in advanced malignancy. Ann Oncol. 2008;19:1969-1974.
1New York Medical College, Valhalla, NY, USA
Corresponding Author:
Sensuke Konno, Department of Urology, New York Medical College
本文部分内容来源网络,如有侵权请联系删除,谢谢。
本文部分内容来源网络,如有侵权请联系删除,谢谢。
编辑:中润世源